Offshore Windparks
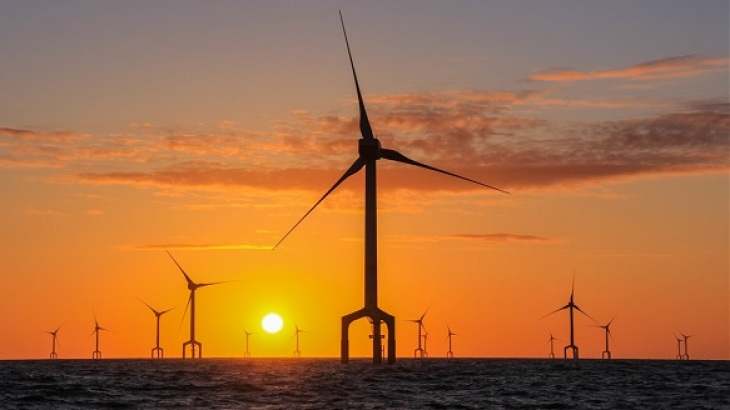
Wind farm in the North Sea (Photo: Matthias Krüger / Hereon)
Offshore Wind Energy Development - A Case for Coastal Research
During the last decade, the North Sea has experienced a considerable increase in offshore wind farm development. At the end of 2021, the capacity in the North Sea has reached a total capacity of 22.5 GW. By 2030, the total offshore wind capacity is expected to be 120 GW and 300 GW until 2050.
This rapid transformation especially of the southern North Sea into an energy seascape bears conflict potential with other use sectors such as fisheries and tourism, while at the same time may compromise nature conservation measures stipulated by national and international legislation.
The Helmholtz-Zentrum Hereon therefore examines the physical, biogeochemical and ecosystem effects of offshore wind farms as well as social and planning aspects.
Various aspects of our research work
- Wind Farm Planning
- Wind Wakes
- Ocean Response to Wind Wakes
- Wind Gusts
- Sediment Wakes
- Ocean Turbulence
- Chemical environmental impact
- Ecosystem Response
- Sociocultural Effects
Wind Farm Planning
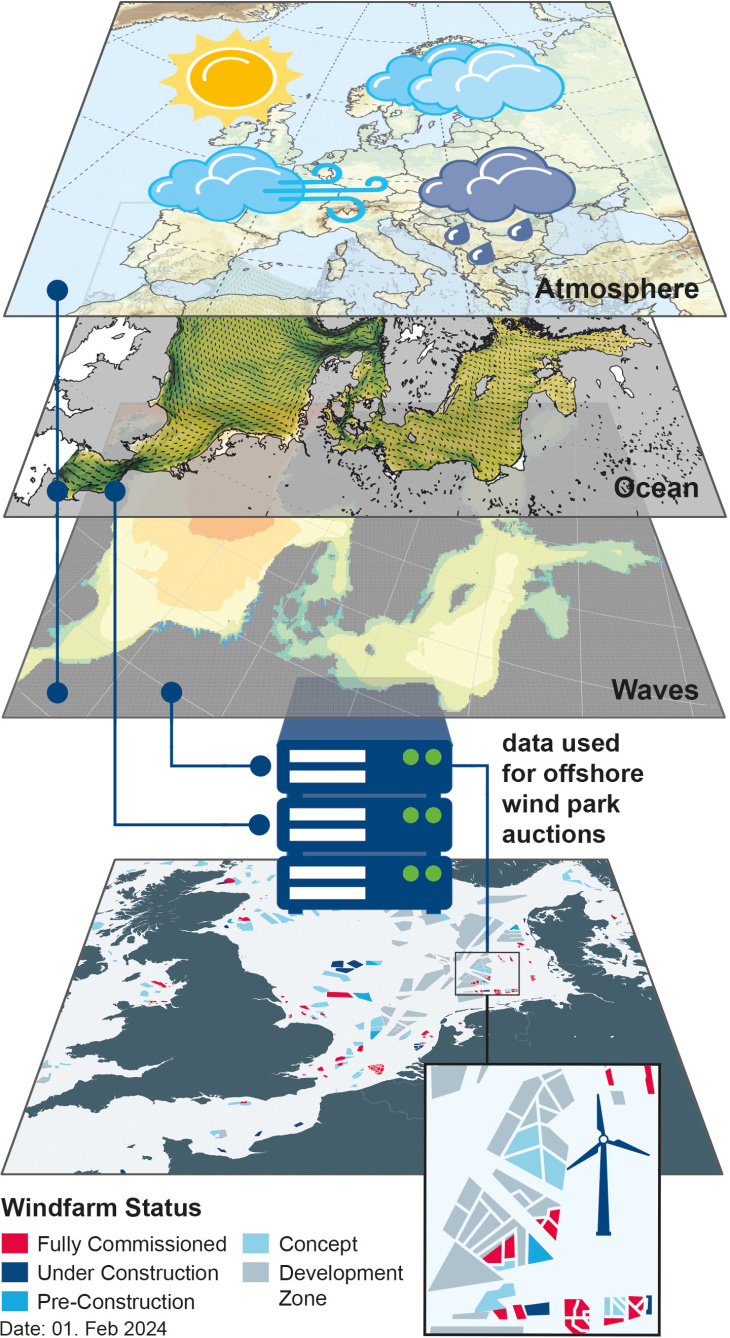
Graphic: A. Kock/ Hereon
Over the last decades, the North Sea has experienced a considerable increase in offshore wind farm development.
Good knowledge of the meteomarine environment is necessary to plan an offshore wind farm. We deliver this database with the Hereon-coastDat data set as illustrated by the figure.
Our high-resolution atmospheric simulations need spatial information on wind farms, the density of the turbines, their hub heights, and power as input.
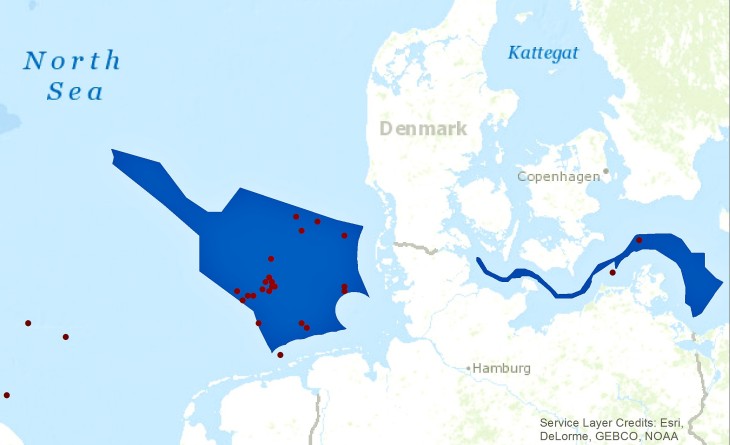
Graphic: U. Kleeberg, E. Meyer/ Hereon
The figure shows those offshore wind farms (red dots) planned with Hereon-coastDat data in the North Sea and Baltic Sea; Germany's exclusive economic zone (EEZ) is shown in blue.
There is considerable interaction between offshore wind farms and the environment, providing challenges and limitations for their operability. The latter has been addressed in several Hereon studies using the coastDat database. For example, a climatology of wind energy over the North Sea was developed also considering potential synergies between different offshore wind farm arrays. In particular, met-ocean data from coastDat is used by nearly all planned and operated offshore wind farms in the German exclusive economic zone for optimization of design and logistics.
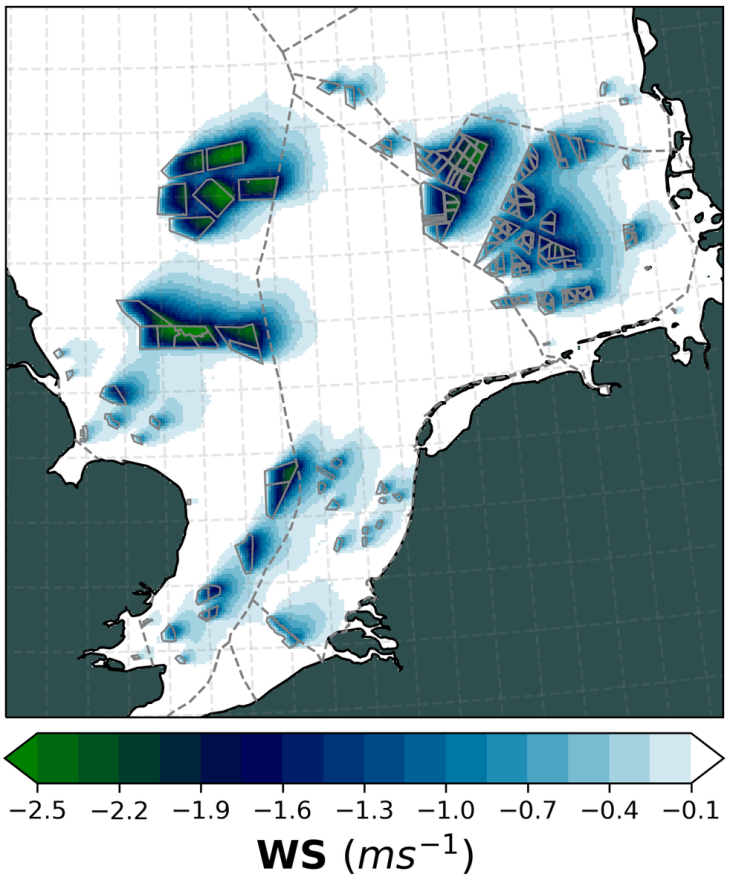
Graphic: N. Akhtar/ Hereon
This figure shows the reduction of windspeed at hub height due to energy extraction by windfarms.
The reduction of windspeed inside of windfarms and for neighbouring wind farms results in the reduction of the capacity factor. This leads to increases in energy production costs and economic losses and has to be taken into account in the planning phase.
In order to assess the impact of major developments in the offshore wind energy sector, we develop technical scenarios based on data from 4C Offshore.
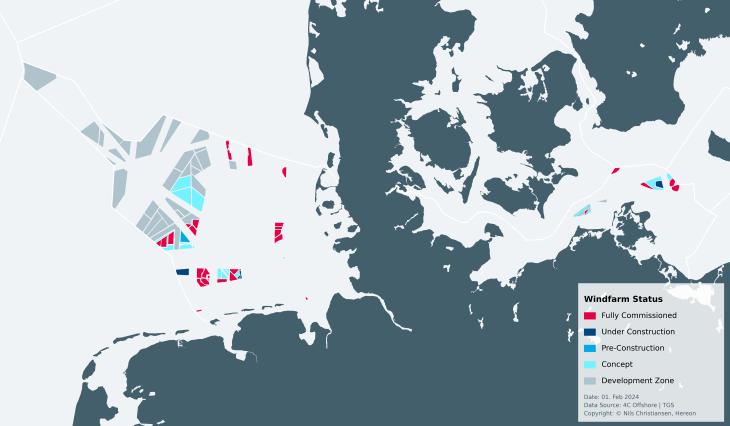
Graphic: Nils Christiansen / Hereon
Existing and planned offshore wind farms, as well as potential development areas in German waters. The different stages of development are indicated by the colors of the wind farm areas.
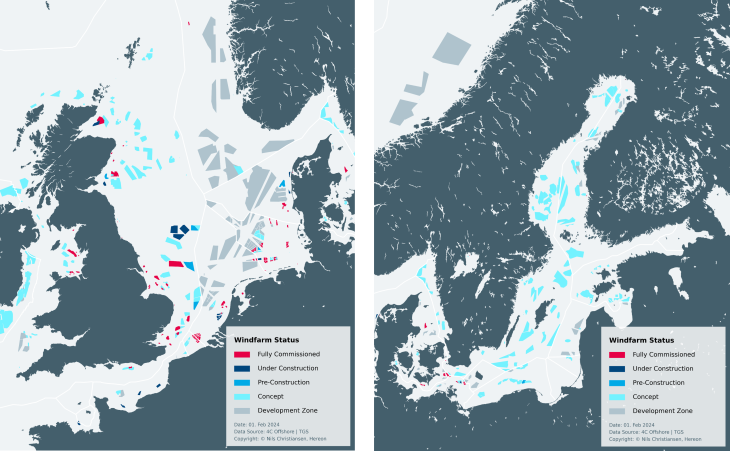
Graphic: Nils Christiansen / Hereon
Expansion status and future projections for offshore wind energy in the North and Baltic Seas. The different stages of development are indicated by the colors of the wind farm areas.
Publications
- Akhtar, N., Geyer, B., Rockel, B., Sommer, P.S., & Schrum, C. (2021): Accelerating deployment of offshore wind energy alter wind climate and reduce future power generation potentials. Sci Rep 11, 11826, doi:10.1038/s41598-021-91283-3
- Geyer, B., Weisse, R., Bisling, P., & Winterfeldt, J. (2015): Climatology of North Sea Wind Energy Derived from a Model Hindcast for 1958-2012. Journal of Wind Engineering and Industrial Aerodynamics, 147, S. 18–29, doi:10.1016/j.jweia.2015.09.005
- Weisse, R., Bisling, P., Gaslikova, L., Geyer, B., Groll, N., Hortamani, M. et al. (2015): Climate Services for Marine Applications in Europe. Earth Perspectives, 2(3), doi:0.1186/s40322-015-0029-0
Wind Wakes
Offshore wind farms (OWFs) extract momentum from the wind field and add turbulence at the same time. The resulting wakes are of practical relevance for different reasons:
1) Wind farms downstream may extract less power from the wind and at the same time the fatigue loading due to turbulence may change.
2) The modified wind field can lead to secondary impacts in the ocean (e.g. circulation, ocean waves or the ecosystem).
3) With a further upscaling of offshore windfarm installations, impacts on larger scale weather features can be anticipated.
Hereon has studied atmospheric wakes using both numerical modelling and satellite observation tools. OWFs can be added in numerical models for the atmosphere using respective parameterization, which take into account different OWF parameters, like the power and thrust coefficients, the rotor diameter, the hub height and the spatial turbine density. A respective simulation for the entire North Sea is shown in Figure 1.
Fig.1: Wind speed anomalies caused by wind farms of 150GW installed capacity modeled with a regional climate model: semi-transparent dark blue (-2 m/s) and brownish (+2 m/s) colors. The colors at the sea surface represent the changes in the latent heat flux. (Animation: A.Elizalde / Hereon, M. Böttinger / DKRZ)
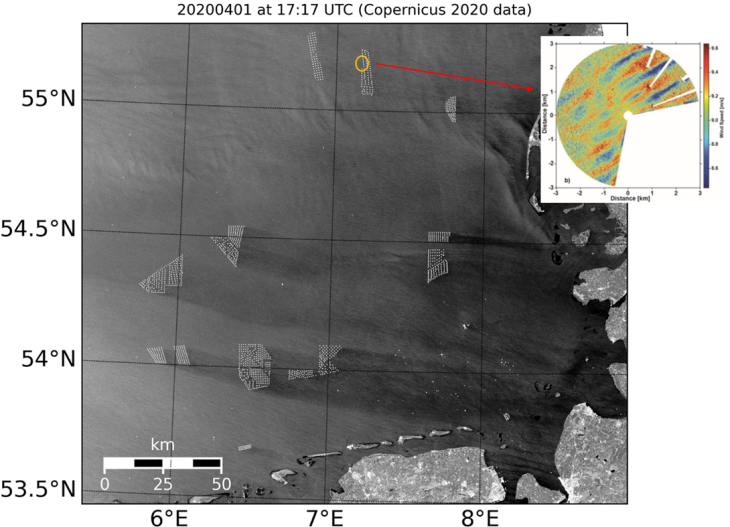
Figure 2: Radiometrically calibrated Sentinel-1A image acquired over the German Bight on April 1st, 2020 at 17:17 UTC (Copernicus Sentinel data 2020). Individual wind turbines can be identified as white dots, which are clustered in different wind parks. The top right corner shows measurements from Hereon’s X-Band radar installed at the research platform FINO-3 (yellow circle).
Atmospheric wakes can also be studied using synthetic aperture radar (SAR) data as provided by the European Sentinel-1 satellites or TerraSAR-X. Figure 2 shows a SAR scene of the German Bight acquired on April 1st, 2020 (Figure 2). The SAR radar signal is correlated with the near surface wind speed, i.e. the brighter areas on the image indicate higher wind speeds and vice versa. It can be seen that the wake lengths can reach up to 100 km and superposition of wakes from different OWFs has been found frequently (Figure 2 and 3). Correlating the satellite observations with measurements from the FINO-1 measurement platform, it could furthermore be shown that the wake lengths is strongly connected to the stability of the atmospheric boundary layer. The wakes tend to be shorter in unstable situations, which typically occur when the air is cooler than the water (Djath et al., 2018; Platis et al., 2020).
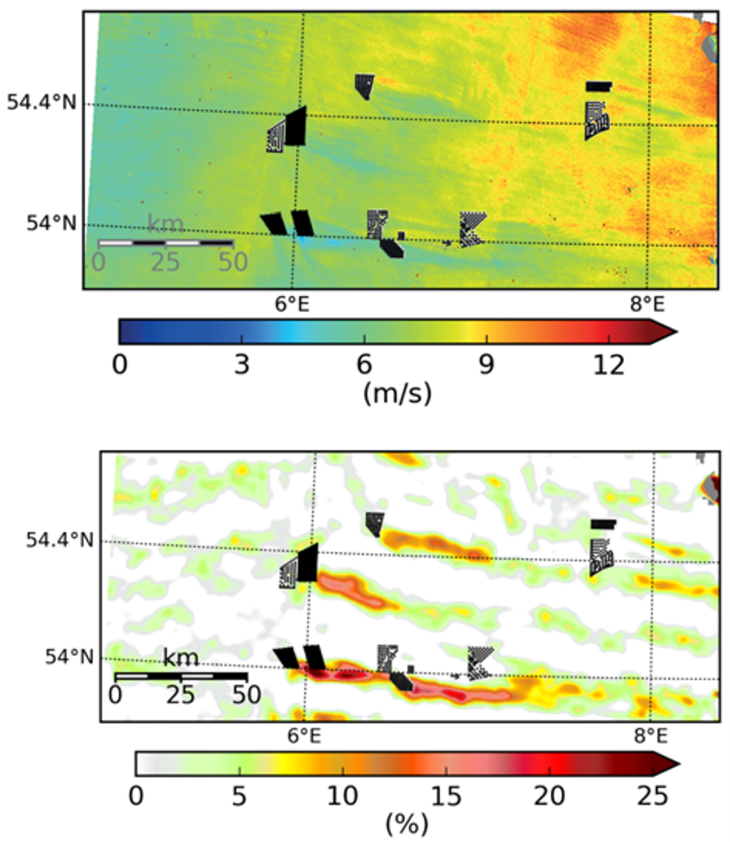
Figure 3: Near surface wind speed derived from a Sentinel-1A scene acquired on 7 April 2017 at 05:49 UTC (top). (bottom) Computed velocity deficit (Djath et al., 2019).
Another field of investigation is the superposition of atmospheric wakes and coastal effects. When the wind is blowing from land towards the sea, the wind speeds typically increase, because the sea surface is smoother than the land and hence the friction is reduced. The OWF wake are typically inside the respective wind speed gradient as shown in Figure 4 (Cañadillas et al., 2022; Djath et al., 2022; Schulz-Stellenfleth et al., 2022).
Wakes were furthermore investigated using Hereon’s X-Band radar installed at the platform FINO-3 (see Figure 2). The radar gives additional information about the temporal evolution of the wind field, e.g. related to gusts and about wind speed shear zones across wakes originating from individual turbines.
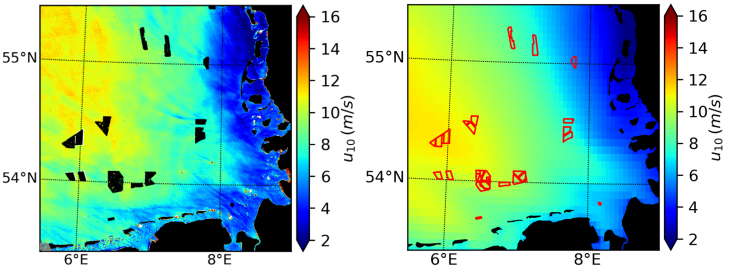
Figure 4: 10 m wind speed from SAR on 6 April 2018 at 17:16UTC (left), DWD collocated 10 m wind (right) showing increase of wind field away from the coast for southeasterly winds (Djath et al., 2022).
Publications
- Akhtar, N., Geyer, B., & Schrum, C. (2022): Impacts of accelerating deployment of offshore windfarms on near-surface climate. Sci Rep 12, 18307 (2022), doi:10.1038/s41598-022-22868-9
- Bärfuss, K., Djath, B., Lampert, A., Schulz-Stellenfleth, J., 2020. Airborne LiDAR Measurements of Sea Surface Properties in the German Bight. IEEE Transactions on Geoscience and Remote Sensing 1–10. https://doi.org/10.1109/TGRS.2020.3017861
- Bärfuss, K., Schulz-Stellenfleth, J., Lampert, A., 2021. The Impact of Offshore Wind Farms on Sea State Demonstrated by Airborne LiDAR Measurement. Mar. Sci. Eng. 9. https://doi.org/10.3390/jmse9060644
- Cañadillas, B., Foreman, R., Barth, V., Siedersleben, S., Lampert, A., Platis, A., Djath, B., Schulz-Stellenfleth, J., Bange, J., Emeis, S., Neumann, T., 2020. Offshore wind farm wake recovery: Airborne measurements and its representation in engineering models. Wind Energy 1–17. https://doi.org/10.1002/we.2484
- Cañadillas, B., Wang, S., Ahlert, Y., Djath, B., Barekzai, M., Foreman, R., & Lampert, A. (2023): Coastal horizontal wind speed gradients in the North Sea based on observations and ERA5 reanalysis data. Meteorologische Zeitschrift (2023), doi:10.1127/metz/2022/1166
- Christiansen, N., Daewel, U., Djath, B., & Schrum, C. (2022): Emergence of Large-Scale Hydrodynamic Structures Due to Atmospheric Offshore Wind Farm Wakes. Front. Mar. Sci. 9:818501, doi:10.3389/fmars.2022.818501
- Djath, B., Schulz-Stellenfleth, J., Canadillas, B., 2018. Impact of atmospheric stability on X-band and C-band Synthetic Aperture Radar imagery of offshore windpark wakes. Journal of sustainable and renewable Energy 10. https://doi.org/10.1063/1.5020437
- Djath, B., Schulz-Stellenfleth, J., 2019. Wind speed deficits downstream offshore wind parks - A new automised estimation technique based on satellite synthetic aperture radar data. Meteorologische Zeitschrift 28, 499–515. https://doi.org/10.1127/metz/2019/0992
- Djath, B., Schulz-Stelllenfleth, J., Cañadillas, B., 2022. Study of Coastal Effects relevant for Offshore Wind Energy using Spaceborne Synthetic Aperture Radar (SAR). Remote Sens. 14. https://doi.org/10.3390/rs14071688
- Emeis, S., Siedersleben, S., Lampert, A., Platis, A., Bange, J., Djath, B., Schulz-Stellenfleth, J., & Neumann, T. (2016): Exploring the Wakes of Large Offshore Wind Farms. Journal of Physics: Conference Series, 753:092014. IOP Publishing, doi:10.1088/1742-6596/753/9/092014
- Lampert, A., Bärfuss, K., Platis, A., Siedersleben, S., Djath, B., Cañadillas, B., Hunger, R., Hankers, R., Bitter, M., Feuerle, T., Schulz, H., Rausch, T., Angermann, M., Schwithal, A., Bange, J., Schulz-Stellenfleth, J., Neumann, T., Emeis, S., 2020. In situ airborne measurements of atmospheric and sea surface parameters related to offshore wind parks in the German Bight. Earth System Science Data 12, 935–946. https://doi.org/10.5194/essd-12-935-2020
- Platis, A., Bange, J., Bärfuss, K., Canadillas, B., Hundhausen, M., Djath, B., Lampert, A., Schulz-Stellenfleth, J., Siedersleben, S., Neumann, T., Emeis, S., 2020. Long-range modifications of the wind field by offshore wind parks – results of the project WIPAFF. Meteorologische Zeitschrift. https://doi.org/10.1127/metz/2020/1023
- Platis, A., Hundhausen, M., Mauz, M., Siedersleben, S., Lampert, A., Bärfuss, K., Djath, B., Schulz-Stellenfleth, J., Canadillas, B., Neumann, T., others, 2021. Evaluation of a simple analytical model for offshore wind farm wake recovery by in situ data and Weather Research and Forecasting simulations. Wind Energy 24, 212–228. https://doi.org/10.1002/we.2568
- Platis, A., Siedersleben, S.K., Bange, J., Lampert, A., Bärfuss, K., Hankers, R., Cañadillas, B., Foreman, R., Schulz-Stellenfleth, J., Djath, B., Neumann, T., Emeis, S., 2018. First in situ evidence of wakes in the far field behind offshore wind farms. Scientific Reports 8, 1--10. https://doi.org/10.1038/s41598-018-20389-y
- Schulz-Stellenfleth, J., Blauw, A., Laakso, L., Mourre, B., She, J., Wehde, H., 2023. Fit-for-Purpose Information for Offshore Wind Farming Applications—Part-II: Gap Analysis and Recommendations. J. Mar. Sci. Eng. 11, 1817. https://doi.org/10.3390/jmse11091817
- Schulz-Stellenfleth, J., Emeis, S., Dörenkämper, M., Bange, M., Canadillas, B., Neumann, T., Schneemann, J., Weber, I., zum Berge, K., Platis, A., Djath, B., Gottschall, J., Vollmer, L., Rausch, T., Barekzai, M., Hammel, J., Steinfeld, G., Lampert, A., 2022. Coastal impacts on offshore wind farms – a review focussing on the German Bight area. Meteorologische Zeitschrift. https://doi.org/10.1127/metz/2022/1109
- She, J., Blauw, A., Laakso, L., Mourre, B., Schulz-Stellenfleth, J., Wehde, H., 2023. Fit-for-Purpose Information for Offshore Wind Farming Applications—Part-I: Identification of Needs and Solutions. J. Mar. Sci. Eng. 11, 1630. https://doi.org/10.3390/jmse11081630
- Siedersleben, S.K., Platis, A., Lundquist, J.K., Djath, B., Lampert, A., Bärfuss, K., Canadillas, B., Schulz-Stellenfleth, J., Bange, J., Neumann, T., Emeis, S., 2020. Turbulent kinetic energy overlarge offshore windfarms observed and simulated by the mesoscale model WRF (3.8.1). Geosci. Model Dev. 13, 249–2020. https://doi.org/10.5194/gmd-13-249-2020
- Siedersleben, S.K., Platis, A., Lundquist, J.K., Lampert, A., Bärfuss, K., Canadillas, B., Djath, B., Schulz-Stellenfleth, J., Bange, J., Neumann, T., Emeis, S., 2018. Evaluation of a Wind Farm Parameterization for Mesoscale Atmospheric Flow Models with Aircraft Measurements. Meteorologische Zeitschrift. https://doi.org/10.1127/metz/2018/0900
- Vicen-Bueno, R., Horstmann, J., Terril, E., Paolo, T. de, & Dannenberg, J. (2013): Real-Time Ocean Wind Vector Retrieval from Marine Radar Image Sequences Acquired at Grazing Angle. J. Atmos. Oceanic Technol., Vol. 30, p. 127–139, doi:10.1175/JTECH-D-12-00027.1
- von Brandis, A., Centurelli, G., Schmidt, J., Vollmer, L., Djath, B., & Dörenkämper, M. (2023): An investigation of spatial wind direction variability and its consideration in engineering models. Wind Energ. Sci., 8, 589–606, doi:10.5194/wes-8-589-2023
Ocean Response to Wind Wakes
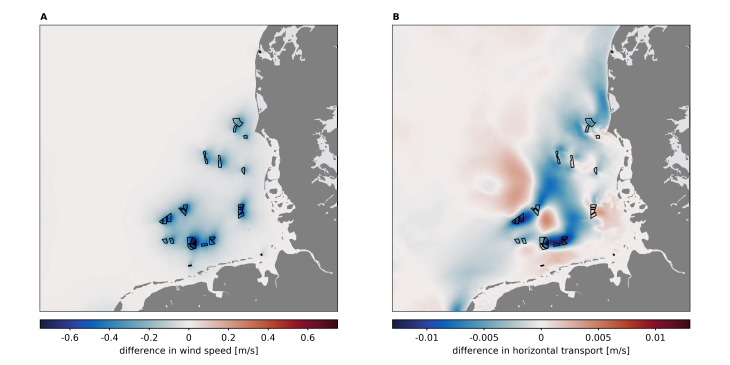
Changes in surface wind speed due to offshore wind farms (A) and their effects on the vertically averaged horizontal volume transport (B) on an annual average.
Harvesting energy from offshore wind resources reduces the wind speed downstream of offshore wind turbines. The resulting wind wakes influence the near-surface winds, which has an impact on wind-driven processes in the ocean such as surface currents, temperature exchange or mixing of the surface layer. For large-scale offshore installations, wind wake effects have been shown to lead to large-scale changes in horizontal transport and density stratification in the ocean, affecting regional hydrodynamics in shelf seas such as the North Sea.
The biogeochemical processes in the marine ecosystems of the lower trophic level are strongly determined by the prevailing physical conditions. Therefore, hydrodynamic disturbances caused by wind currents have a direct impact on ecosystem dynamics and influence, for example, vertical nutrient transport, primary production or local biomass.
Regional and high-resolution models are used to investigate the effects of a changing wind field on hydro- and ecosystem dynamics in the North Sea. These include coupled physical-biogeochemical models as well as flexible models with unstructured grids.
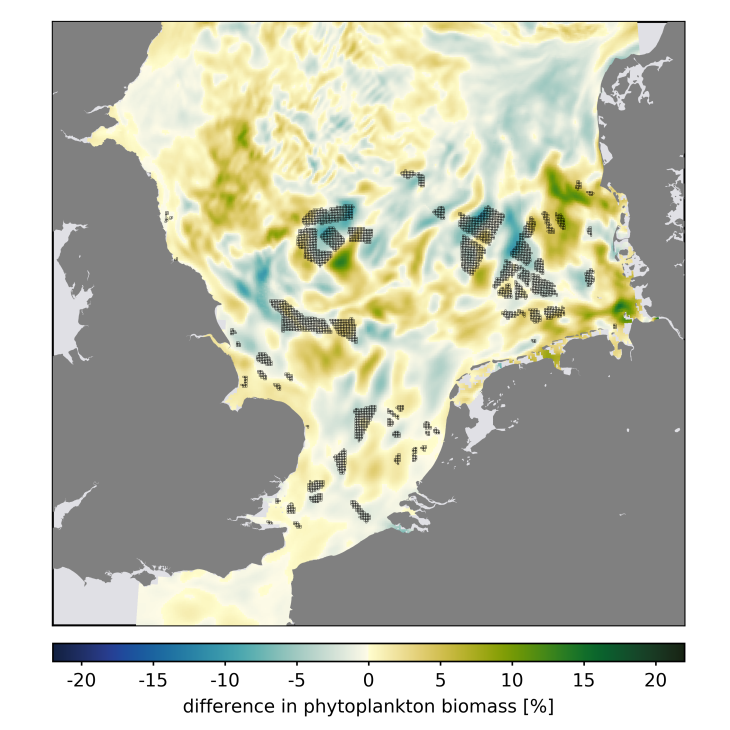
Relative changes in the annually and vertically averaged phytoplankton biomass due to atmospheric turbine currents
Publications
- Christiansen, N., Daewel, U., Djath, B., and Schrum, C. 2022. Emergence of Large-Scale Hydrodynamic Structures Due to Atmospheric Offshore Wind Farm Wakes. Frontiers in Marine Science, 9. doi: 10.3389/fmars.2022.818501
- Christiansen, N., Daewel, U., and Schrum, C. 2022. Tidal mitigation of offshore wind wake effects in coastal seas. Frontiers in Marine Science, 9. doi: 10.3389/fmars.2022.1006647
- Daewel, U., Akhtar, N., Christiansen, N., and Schrum, C. 2022. Offshore wind farms are projected to impact primary production and bottom water deoxygenation in the North Sea. Communications Earth & Environment, 3: 292. doi: 10.1038/s43247-022-00625-0
Wind Gusts
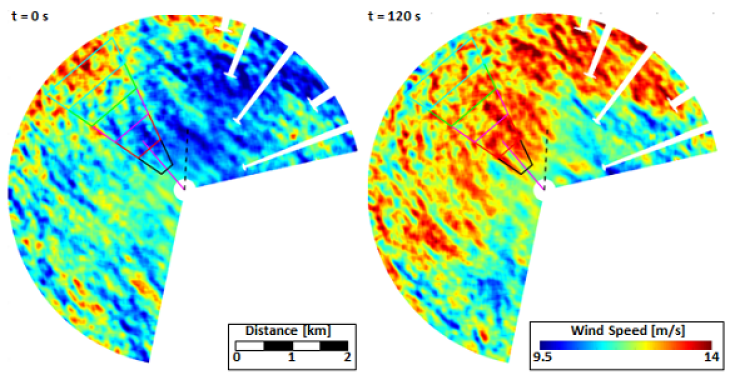
Surface radar retrieved wind fields (120 s apart in time) of a wind gust propagating towards the offshore platform Fino-3 in the German Bight (Graphic: Jochen Horstmann / Hereon)
For an improved predictive control of offshore wind farms, Hereon has developed a short-term (30 to 60 s) wind speed and gust prediction system. Wind fields are retrieved from marine radar image sequences to identify wind gusts and estimate their size and propagation speed and direction.
Sediment Wakes
To study vortices generated by the piles of offshore wind farms, an unstructured grid ocean model (SCHISM) was applied (Grashorn and Stanev, 2016). It was shown that the model can reproduce suspended matter concentration features, which were previously observed in optical satellite imagery.
Introducing turbulence in the water offshore wind farm piles can have a significant influence on the sediment dynamics. Higher turbulent energy behind the piles causes increased vertical mixing and hence can lead to higher concentrations of sediment near the sea surface.
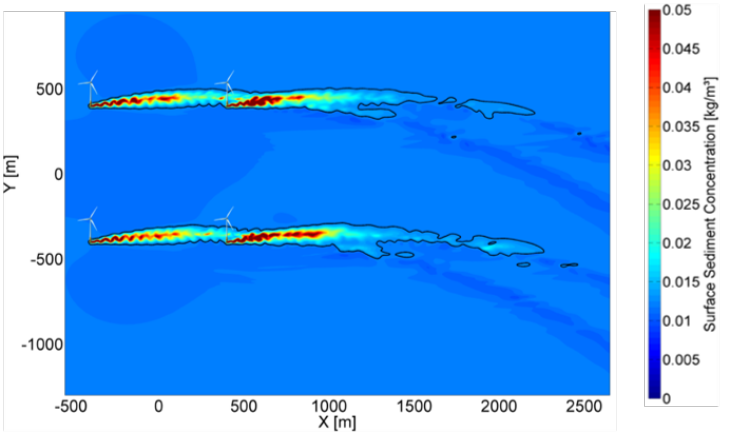
Graphic: Sebastian Grashorn/ Hereon
Snapshots for surface sediment concentration of suspended particle matter for a model basin with a depth of 21.6 m.
This effect was successfully simulated using the unstructured grid model SCHISM run at Hereon (Grashorn and Stanev, 2016). The model is well suited for this application because it can resolve small-scale processes in the vicinity of the piles using a finer computational mesh in that region. The vortices of increased surface sediment concentrations shown in the image are driven by tidal currents typical for the German Bight.
The impact of offshore wind farms on sediment dynamics extends far beyond the local-scale erosion/deposition patterns. It may also impact ecosystem functioning and carbon sequestration in sediments. Vessel traffic in general, and use of bottom-contacting fishing gears especially, is usually restricted or banned within offshore wind farms (OFWs). A substantial increase in conflict potential between OWFs and fisheries in the North Sea is therefore to be expected during the next decades, with several riparian nations' plans for the construction of extensive offshore renewable energy infrastructure. For this scenario, wind farms in the 4C Offshore database (https://www.4coffshore.com/windfarms/, last access: 2 May 2023) are considered, excluding those where the project status is classified as “canceled”, “decommissioned”, or “failed proposal”. We adopted the parameterizations of Christiansen et al. (2022a, b, 2023), which were developed and validated for the North Sea, to account for the wind wake and pile effects in particle transport.
Our results show that the OWFs exert a relatively small impact on overall particulate organic carbon sedimentation and burial, owing to the limited overlap between trawling grounds, OWFs, and areas of carbon deposition, as well as the mitigating factor of reduced wind speed, which decreases wind stress and thereby natural current resuspension within and downwind of OWFs. Strong local effects do occur in the model, and the wind wakes and pile turbulence production cause the impacts to extend to considerable distances from the OWFs themselves. A holistic assessment of OWF impacts on sediment carbon should consider the carbon loss due to seafloor disturbance during construction and decommissioning, as well as secondary effects, such as the colonization of organisms at the foundations of wind turbines and wind wake impacts on the ecosystem structure. Using an ecosystem model that considers wind wake effects, Daewel et al. (2022) simulated local increases in sedimentary carbon of up to 10 % after 1 year but only a slight net increase of 0.2 % for the entire North Sea. Though our OWF scenario shows a slight decrease in sedimentary particulate organic carbon, this is primarily due to the trawling effort redistribution, whereas the wind wake effect shows a similar sign and magnitude to Daewel et al. (2022). Heinatz and Scheffold (2023) estimated a net increase in sediment OC storage at OWFs in the southern North Sea on the order of 1000 kt throughout a 20-year life cycle, or 50 kt yr−1. This estimate is 1 order of magnitude greater than our estimated reduction. Overall, a comparison of these budgets implies a net positive impact of OWFs on organic carbon storage, with local impacts at the foundations outweighing the large-scale impacts that redistribute organic carbon in the far field. Plans for areas of OWF development have expanded rapidly during recent years and continue to evolve quickly, making an increased impact on organic carbon in the future likely.
Impacts of wake and pile effects
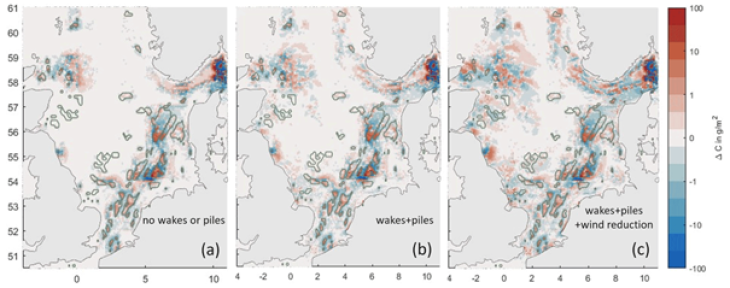
Graphic: Wenyan Zhang/ Hereon
Impacts of wake and pile effects in offshore wind farms on particulate organic carbon redistribution. Colors show the average end-of-year difference in total sediment organic carbon between the OWF scenario and the baseline simulation for the year 2000. Panel (a) shows impact due to trawling redistribution alone, panel (b) additionally includes wind speed reduction in the wakes of and enhanced turbulence inside of OWFs, and panel (c) additionally includes wind speed reduction inside of the OWFs.
Publications
- Christiansen, N., Daewel, U., Djath, B., and Schrum, C.: Emergence of Large-Scale Hydrodynamic Structures Due to Atmospheric Offshore Wind Farm Wakes, Front. Mar. Sci., 9, 818501, https://doi.org/10.3389/fmars.2022.818501, 2022a
- Christiansen, N., Daewel, U., and Schrum, C.: Tidal mitigation of offshore wind wake effects in coastal seas, Front. Mar. Sci., 9, 1006647, https://doi.org/10.3389/fmars.2022.1006647, 2022b
- Christiansen, N., Carpenter, J.R., Daewel, U., Suzuki, N., and Schrum, C.: The large-scale impact of anthropogenic mixing by offshore wind turbine foundations in the shallow North Sea, Front. Mar. Sci., 10, 1178330, https://doi.org/10.3389/fmars.2023.1178330, 2023
- Daewel, U., Akhtar, N., Christiansen, N., and Schrum, C.: Offshore wind farms are projected to impact primary production and bottom water deoxygenation in the North Sea, Commun. Earth Environ., 3, 292, https://doi.org/10.1038/s43247-022-00625-0, 2022.
- Grashorn, S., & Stanev, E.V. (2016): Kármán Vortex and Turbulent Wake Generation by Wind Park Piles. Ocean Dynamics, 66:1543–1557, doi:10.1007/s10236-016-0995-2
- Heinatz, K. and Scheffold, M. I. E.: A first estimate of the effect of offshore wind farms on sedimentary organic carbon stocks in the Southern North Sea, Front. Mar. Sci., 9, 1068967, https://doi.org/10.3389/fmars.2022.1068967, 2023.
- Porz, L., Zhang, W., Christiansen, N., Kossack, J., Daewel, U., & Schrum, C. (2024): Quantification and mitigation of bottom-trawling impacts on sedimentary organic carbon stocks in the North Sea. Biogeosciences, 21, 2547–2570, doi:10.5194/bg-21-2547-2024
Ocean Turbulence
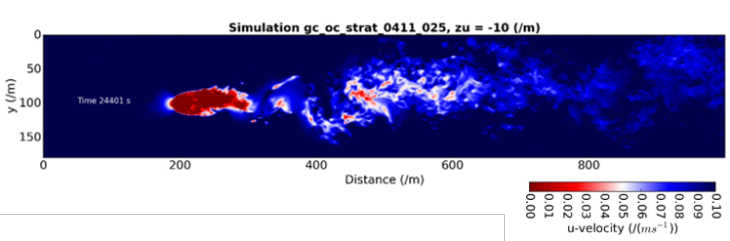
Turbulent velocity field in the wake of a cylindrical offshore wind farm structure. This is a snapshot from a Large Eddy Simulation looking from above on the water surface, with the current moving from left to right past the circular structure. Blue indicates a strong current, and red a weak current (Graphic: Jeff Carpenter / Hereon)
Ocean turbulence is caused by the interaction of tidal currents and the OWF structures. It provides an additional mixing potential for the seasonal stratification that forms throughout large areas of the German EEZ. In this way, large-scale OWF constructions can have a significant impact on North Sea stratification.
Current work is focused on understanding and quantifying the localised mixing process of a single OWF foundation using Large Eddy Simulations and in situ measurements. Initial results suggest that the low thermocline turbulence is expected to be enhanced by OWFs.
In addition to the high-resolution Large Eddy Simulations, regional ocean modeling is used to understand the impact of the mixing processes on North Sea stratification. By parameterizing the drag and turbulence generated from monopiles, recent work showed that foundation effects can extend far beyond turbine locations and influence summer stratification over large areas in the German Bight.
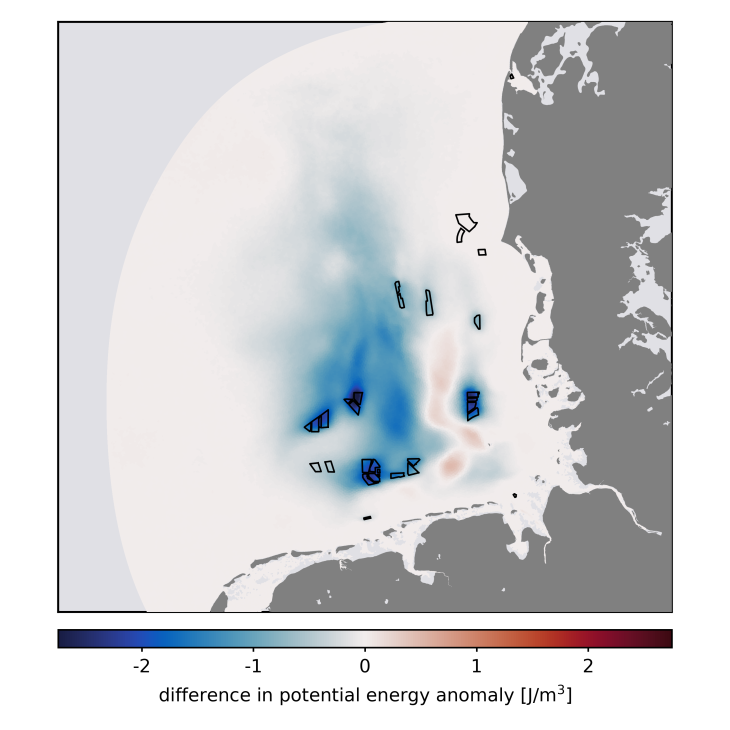
Changes in summer stratification due to induced turbulence from offshore wind farm structures in the German Bight. The potential energy anomaly is a measure for the intensity of the vertical density stratification of the water column.
Publications
- Carpenter, J.R., Merckelbach, L., Callies, U., Clark, S., Gaslikova, L., & Baschek, B. (2016): Potential Impacts of Offshore Wind Farms on North Sea Stratification. PLoS ONE, 11(8), e0160830, doi:10.1371/journal.pone.0160830
- Christiansen, N., Carpenter, J. R., Daewel, U., Suzuki, N., and Schrum, C. 2023. The large-scale impact of anthropogenic mixing by offshore wind turbine foundations in the shallow North Sea. Frontiers in Marine Science, 10. doi: 10.3389/fmars.2023.1178330
- Floeter, J., Beusekom, J.E.E. van, Auch, D., Callies, U., Carpenter, J.R., et al. (2017): Pelagic Effects of Offshore Wind Farm Foundations in the Stratified North Sea. Progress in Oceanography, 156, 154–173, doi:10.1016/j.pocean.2017.07.003
- Schultze, L.K.P., Merckelbach, L., & Carpenter, J.R. (2017): Turbulence and Mixing in a Shallow Stratified Shelf Sea from Underwater Gliders. Journal of Geophysical Research -- Oceans, 122, doi:10.1002/2017JC012872
Chemical environmental impact
The North Sea is surrounded by highly populated and industrialised countries. The anthropogenic pressure on the marine ecosystem is undisputed: pollutants from inland areas enter the sea via rivers and humans have long since taken over the sea itself as a space for use. Shipping, fishing, dumping and, more recently, the production of wind energy all take place in the North Sea. In addition, there are plans to produce green hydrogen and derived products such as ammonia, methanol and synthetic fuels offshore in the near future. These anthropogenic activities carry the risk of additional pollutant inputs. Valid analytical methods are therefore needed for targeted monitoring and for source differentiation of new, but also already known, pollutants, as well as for determining a chemical baseline to allow a further impact assessment.
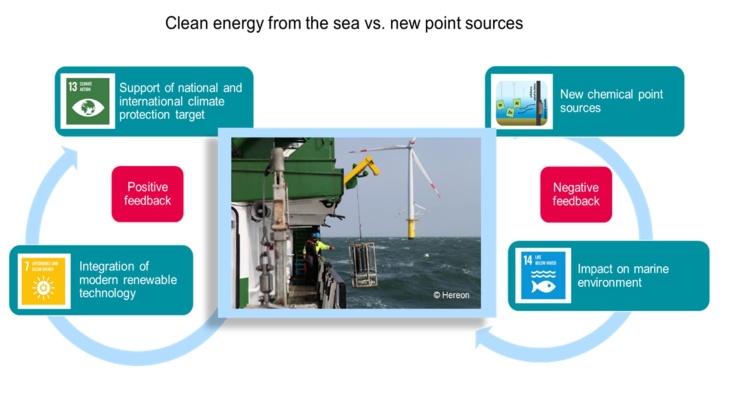
Figure 1 Offshore wind farms are at the centre of the conflict between the SDGs that call for the generation of renewable energies (SDG #7 and SDG #13), while at the same time having an impact on the marine environment as new point sources of emissions (SDG #14). (© Lange et al., 2023)
In particular, the corrosion protection of the large offshore wind steel structures, which is in direct contact with the marine environment and is unavoidable, is a source of emissions. Galvanic anodes even emit their alloy components aluminium, zinc and indium by design, but also other metals they contain such as gallium, cadmium and lead. These anodes are designed to corrode instead of the structural steel, resulting in a continuous emission of metals (e.g. >2000 kg Al anode material per pile for the 25-year lifespan) into the marine environment.
In cooperation with the Federal Maritime and Hydrographic Agency, offshore wind farms in the German North and Baltic Seas have been sampled regularly since 2016. Water and sediment samples are analysed for metals. In addition, isotope and elemental ratios are determined to draw further conclusions about the origin of the measured metals.
In Figure 2, data from 2020 and 2021 are shown as distribution maps. For each year, the gadolinium anomaly is shown as a tracer for riverine inputs, the indium concentration as a tracer for galvanic anodes, and drift trajectories are shown that represent the trajectory of the sampled water body for the five days prior to sampling. By combining these three parameters, the increased indium concentration measured in 2020 in the northern wind farm can be attributed to the use of galvanic anodes in offshore wind farms. However, this phenomenon is not visible in 2021. This is due to, for example, different mixing and greater influence of coastal waters at the time of sampling.
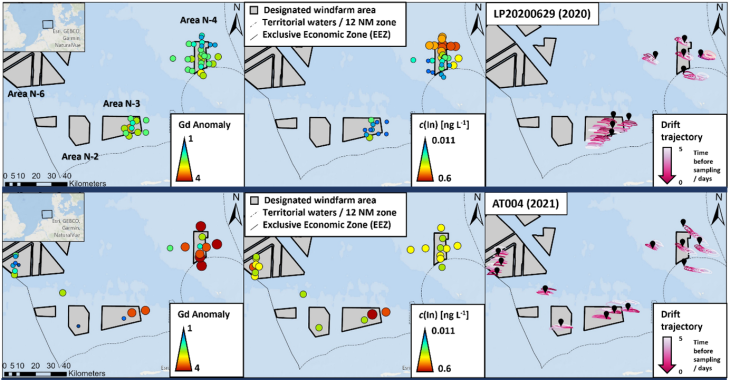
Figure 2 Spatial distribution (from left to right) of the gadolinium anomaly, the indium concentration and the backward trajectory of the water masses for selected sampling points in and around offshore wind farms in the German Bight in 2020 (top) and 2021 (bottom) (manuscript submitted for publication; data: https://doi.org/10.1594/PANGAEA.963880, https://doi.pangaea.de/10.1594/PANGAEA.973612).
Sampling seawater only provides a snapshot of dissolved metal concentrations. Changes in current conditions due to storms, for example, also alter the dissolved metal content. Sampling sediment, on the other hand, provides information about long-term changes in pollution. Figure 3 shows the mass fractions of indium as boxplots in sediments from offshore wind farms. Annual and regional differences are detectable. The data do not yet indicate an observable increase or accumulation of metal mass fractions in sediments from offshore wind farms. However, in a dynamic system like the North Sea with its rather coarse-grained sediments, it could take longer for a measurable change in metal mass fractions to become apparent. Therefore, the data sets collected are an important reference point for the future monitoring of offshore wind-induced emissions and help to close the knowledge gap in background data, particularly for indium and gallium in the North Sea.
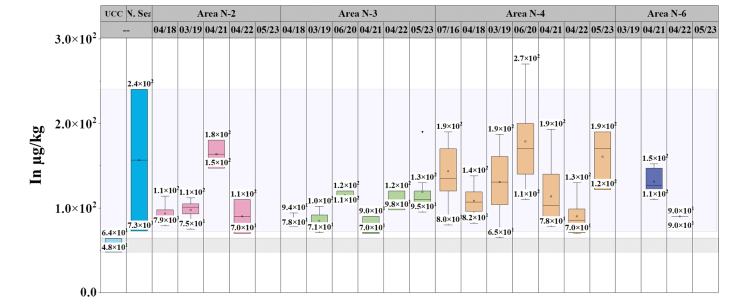
Figure 3 Indium mass fractions in sediments (<20 µm fraction) in offshore wind farms in the German Bight per wind farm area as a time series from 2016 to 2023. Overall, there are some large differences between the areas and locally elevated indium mass fractions have been found (e.g. Area N-4 in 2020). So far, however, no clear temporal trends are recognisable. (from Ebeling et al., 2023; extended by the values from 2023)
OffChEm & OffChEm II: Chemical emissions from offshore wind farms – Potential impacts on the marine environment and their evaluation OffChEm
H2Mare hydrogen offshore flagship project H2Mare
Publications
- Reese, A., Voigt, N., Zimmermann, T., Irrgeher, J., Pröfrock, D., 2020. Characterization of alloying components in galvanic anodes as potential environmental tracers for heavy metal emissions from offshore wind structures. Chemosphere 257, 127182. https://doi.org/10.1016/j.chemosphere.2020.127182
- Ebeling, A., Wippermann, D., Zimmermann, T., Klein, O., Kirchgeorg, T., Weinberg, I., Hasenbein, S., Plaß, A., Pröfrock, D., 2023. Investigation of potential metal emissions from galvanic anodes in offshore wind farms into North Sea sediments. Marine Pollution Bulletin 194, 115396. https://doi.org/10.1016/j.marpolbul.2023.115396
- Lange, M., Cabana, D., Ebeling, A., Ebinghaus, R., Joerss, H., Rölfer, L., Celliers, L., 2023. Climate-smart socially innovative tools and approaches for marine pollution science in support of sustainable development. Cambridge Prisms: Coastal Futures 1, e23. https://doi.org/10.1017/cft.2023.11
- Hildebrandt, L., Fischer, M., Klein, O., Zimmermann, T., Fensky, F., Siems, A., Zonderman, A., Hengstmann, E., Kirchgeorg, T., Pröfrock, D., 2024. An analytical strategy for challenging members of the microplastic family: Particles from anti-corrosion coatings. Journal of Hazardous Materials 470, 134173. https://doi.org/10.1016/j.jhazmat.2024.134173
- Bedulina, D., Korez Lupše, Š., Hildebrandt, L., Duan, Y., Klein, O., Primpke, S., Bock, C., Krause, S., Czichon, S., Pröfrock, D., Gerdts, G., Lannig, G., 2024. Effect of Particles from Wind Turbine Blades Erosion on Blue Mussels Mytilus Edulis. Science of the Total Environment 957, 177509. https://doi.org/10.1016/j.scitotenv.2024.177509
Ecosystem Response
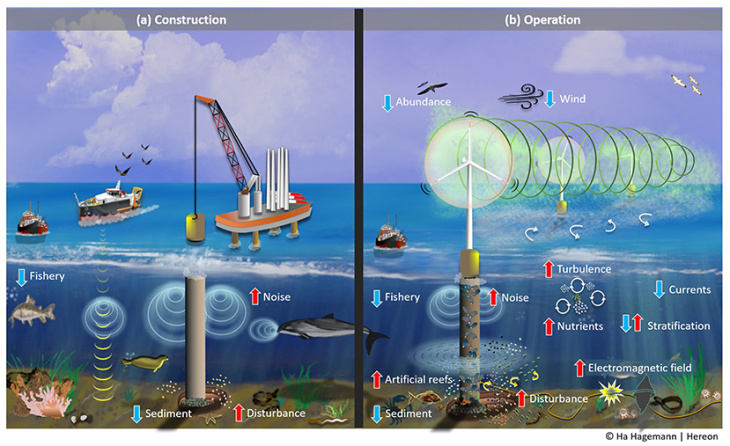
Offshore wind turbines have effects on the environment and ecosystems during (a) their construction, and (b) when they are in operation. Blue arrows represent a decrease in a specific process and red arrows indicate an increase. (Benkort, 2024)
OWF effects on marine biology have been reported for different aspects of the marine ecosystem (see Figure).
The prevailing physical conditions strongly determine biogeochemical processes in the lower trophic marine ecosystem. As a result, the hydrodynamic disturbances caused by wind wakes directly affect ecosystem dynamics and influence, for example, vertical nutrient transport, primary production, or local biomass.
Regional and high-resolution models are used to study the effects of a modified wind field on the North Sea's hydro- and ecosystem dynamics, including coupled physical-biogeochemical models and flexible unstructured-grid models.
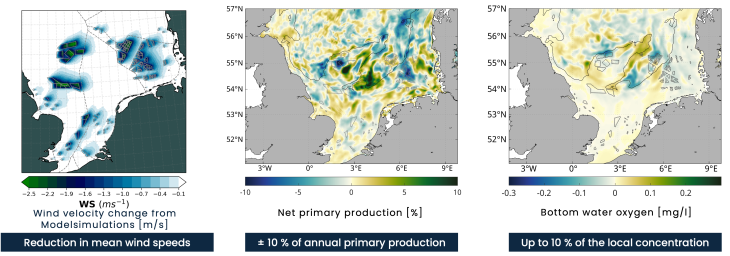
Annual mean changes in wind speed, net primary production and bottom water oxygen concentration, estimated for 120GW OWF scenario, see Akhtar et al. 2022 (left) and Daewel et al. 2022(middle/right).
In a model scenario simulation, where we assumed 120 GW installation in the southern North Sea (Figure), we simulated the response of the marine ecosystem to OWF generated wind-wakes. The results indicating a large-scale restructuring of the marine ecosystem with local changes of up to 10%, highlighting that the effects on the ecosystem reach far beyond the extend of the offshore wind farms themselves.
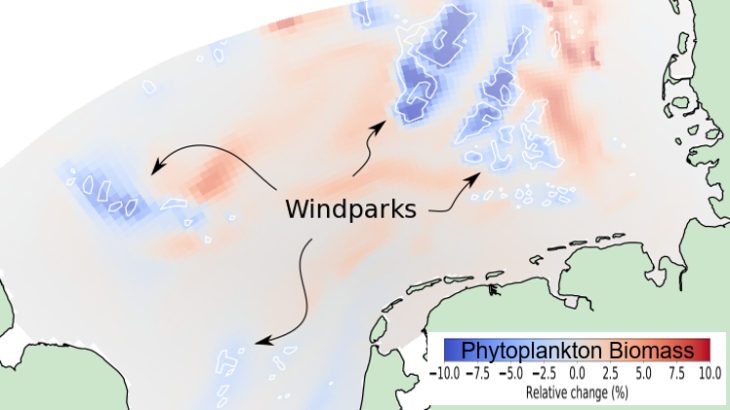
Simulated changes of phytoplankton biomass expected from epistructural filtration by the blue mussel mytilus edulis on newly created habitats on offshore wind farm piles. (Graphic: Carsten Lemmen / Hereon)
The build-up of offshore wind farms provides new hard surfaces in the water column that is a preferred habitat of the blue mussel (mytilus edulis). These blue mussels filter phytoplankton (algae) out of the water thus make the water clearer.
Computer simulations of the southern North Sea indicate how much clearer the water could be if all planned offshore wind farm piles were settled by blue mussels. An estimated 10 % of the algae are removed by the filtration from these epistructural blue mussels in or near the wind farms, and up to 10 % more algae are expected due to secondary effects, such as more nutrient availability leeward of the offshore wind farm areas.
Publications
- Akhtar, N., Geyer, B., and Schrum, C. 2022. Impacts of accelerating deployment of offshore windfarms on near-surface climate. Scientific Reports, 12: 1–16. Nature Publishing Group UK. doi:10.1038/s41598-022-22868-9.
- Benkort, D., Christiansen, N., Ho-Hagemann, H. T. M., Daewel, U., and Gilles, A. 2024. How Do Offshore Wind Farms Affect the Ocean? Frontiers for Young Minds, 12. https://doi.org/10.3389/frym.2024.1336535
- Daewel, U., Akhtar, N., Christiansen, N., and Schrum, C. 2022. Offshore wind farms are projected to impact primary production and bottom water deoxygenation in the North Sea. Communications Earth & Environment, 3: 292. doi: 10.1038/s43247-022-00625-0
- Slavik, K., Lemmen, C., Zhang, W., Kerimoglu, O., Klingbeil, K., & Wirtz, K.W. (2018): The Large Scale Impact of Offshore Windfarm Structures on Pelagic Primary Production in the Southern North Sea. Hydrobiologia, pp 1-19, doi:10.1007/s10750-018-3653-5
Marine use conflicts and sociocultural effects
The expansion of offshore wind energy from currently up to 70 GW in the German North Sea and up to 300 GW in the entire North Sea Region leads to considerable competition for space with other uses, in particular nature conservation and fisheries. This is associated with conflicts between the affected user groups, both in terms of their respective interests and their respective mental images of the future of the marine space. Fishers as revealed in a set of interviews fear losing traditional fishing grounds, while nature conservation protagonists indicated in interviews and workshops concerns about changes to natural habitats, impacts on protected species as well as effects on protected areas and marine ecosystems per se.
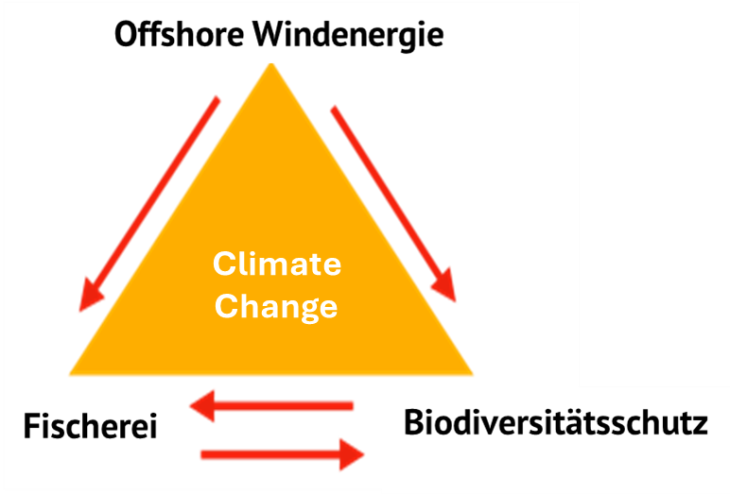
Triangle of key actors for futures use in the German North Sea (Graphics: Jürgen Schaper, Andreas Kannen / Hereon)
In an inter- and transdisciplinary context, the social scientists at Hereon are analyzing conflicts of use, but also the specific perceptions of the marine space of different user groups, their adaptive capacities to change and translate this into approaches for Maritime Spatial Planning (MSP).
Earlier work at Hereon illustrated specifically arguments of local coastal population related to offshore wind farm development. A comparison of these arguments with the respondent's personal values, beliefs, and perception of the area, reveal different patterns of argumentation requiring various differentiated communication strategies by planners and investors within planning and approval processes.
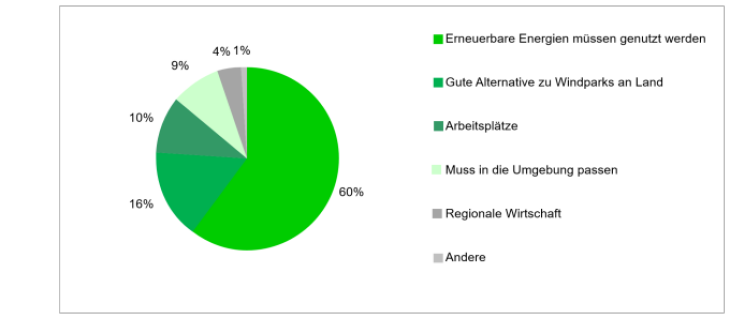
Arguments mentioned by local residents in support of offshore wind farms along the Wadden Sea coast in Schleswig-Holstein. (Graphic: Kira Gee, Andreas Kannen / Hereon)
Analyses of socio-cultural perspectives of offshore wind farms thus support a better understanding of resistance, acceptance and support by residents and particular actor groups.
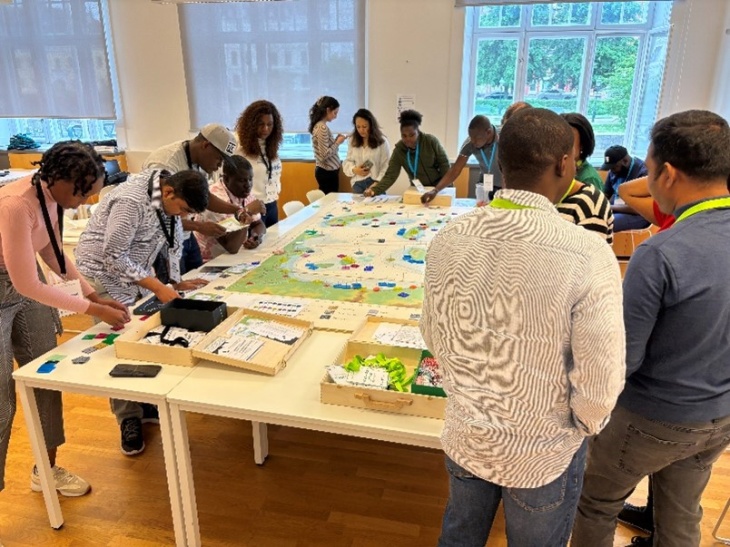
Training course for Maritime Spatial Planning at the World Maritime University, Malmoe. (Photo: Andreas Kannen / private)
Based on these results, Hereon researchers have been regularly analyzing the volatile political, legal and planning contexts. Results are transposed by Hereon researchers into methodological proposals to improve marine planning and management, such as by outlining a concept for recognizing culturally significant areas. In collaboration with other research groups, this is supported by analyses of adaptation capacities, e.g. for fisheries, and currently in the CoastalFutures project by transdisciplinary dialogue forums with various stakeholder groups on options, opportunities and constraints for multi-use concepts for the marine space and co-uses of specific marine places. The knowledge gained in these analyses is integrated into international research and advice of the International Council for the Exploration of the Sea (ICES) and in international trainings in Maritime Spatial Planning (MSP).
Publications
- Zaucha, J, Gee, K, Ramieri, E, Neimane, L, Alloncle, N, Blažauskas, N, Calado, H, Cervera-Núñez, C, Kuzmanov, VM, Stancheva M, Witkowska, J, Sigrid Eskeland Schütz, S, Zapatero, JR, Ehler, CN (2024): Implementing the EU MSP Directive: Current status and lessons learned in 22 EU Member States, Marine Policy, 171 (2025), https://doi.org/10.1016/j.marpol.2024.106425
- Stelzenmüller, V., Letschert, J., Blanz, B., Blöcker, A.M., Claudet, J., Cormier, R., Gee, K., Held, H., Kannen, A., Kruse, M.,Rambo, H., Schaper, J., Sguotti, C., Stollberg, N., Quiroga, M., Möllmann, C. (2024): Exploring the adaptive capacity of a fisheries social-ecological system to global change, Ocean & Coastal Management, Volume 258, 2024, 107391, ISSN 0964-5691, https://doi.org/10.1016/j.ocecoaman.2024.107391
- Gee, K., Arndt, P., Dahmen, M., Geitel, N.-K., Hunke, A., Käppeler, B., Plug, D., Scheffler, U., & Trümpler, K. (2024): A new maritime spatial plan for the German EEZ: Navigating multiple aspirations and spatial developments. Marine Policy, Vol 161, 106006, doi:10.1016/j.marpol.2024.106006
- Kruse, M., Letschert, J., Cormier, R., Rambo, H., Gee, K., Kannen, A., Schaper, J., Möllmann, C-, & Stelzenmüller, V. (2024): Operationalizing a fisheries social-ecological system through a Bayesian belief network reveals hotspots for its adaptive capacity in the southern North Sea. Journal of Environmental Management, Vol 357, 120685, doi:10.1016/j.jenvman.2024.120685
- Gee, K. and Mikkelsen, E. (2023): Understanding different types of conflicts and coexistence in marine spatial planning (MSP). ICES Cooperative Research Reports Vol. 357. 52 pp. https://doi.org/10.17895/ices.pub.24598734
- Cormier, R., & Kannen, A. (2019): Managing Risk Through Marine Spatial Planning. In: Zaucha, J., & Gee, K. (eds): MaritimeSpatial Planning. Palgrave Macmillan, Cham, https://link.springer.com/chapter/10.1007/978-3-319-98696-8_15
- Gee, K., Kannen, A., Adlam, R., Brooks, C., Chapman, M., Cormier, R., Fischer, C., Fletcher, S., Gubbins, M., Shucksmith, R., & Shellock, R. (2017): Identifying Culturally Significant Areas for Marine Spatial Planning. Ocean and Coastal Management, 136, pp. 139-147, doi:10.1016/j.ocecoaman.2016.11.026
- Gee, K. (2013): Trade-offs Between Seascape and Offshore Wind Farming Values: An Analysis of Local Opinions Based on a Cognitive Belief Framework (pdf) PhD thesis University of Goettingen, p. 245